The first thing you see as you enter Ron Saporta’s office is the large solar panel that serves as a table top. The panel isn’t hooked up to generate electricity, but it reminds Saporta – and his visitors – of a central part of his mission these days: to help steer this almost 200-year-old institution to a low-carbon future.
Saporta, who is U of T’s chief operating officer for property services and sustainability, is keenly aware of the scope of the challenge. He likes to point out that if the university were a city, it would rank among the 40 most populous in Canada. Many of the buildings on the St. George campus were constructed more than a century ago, when fire was the main concern – not energy efficiency. Updating these buildings, many of which are heritage listed, to current green standards will require a large upfront investment.
The payoff for the university will come in the form of lower energy bills and reduced greenhouse gas emissions. In 2018, U of T made a commitment to cut its carbon footprint to 37 per cent below 1990 levels by 2030 – even though its three campuses have undergone (and will continue to see) significant growth. The majority of the planned reduction will come in the next five years.
To meet this goal, Saporta and his team examined how the university consumes, produces and distributes energy. Their Low-Carbon Action Plan, released last fall, identifies ways to reduce greenhouse gas emissions at each of these stages (what Saporta calls a “life cycle” approach) and evaluates each potential project to ensure it benefits the university over the long term. “If we use less energy, we spend less money,” he says. “This makes for a strong business case.”
The university’s data centre is a prime example. The array of computer servers gives off so much heat that parts of the building require constant air conditioning, even in winter. So later this year, the university will install a heat-recovery system to redistribute that energy and help keep the building warm during winter. Estimated savings: $175,000 a year, and 1,000 tonnes of carbon dioxide emissions.
Because of U of T’s strength in research, Saporta envisions using the university as a “living laboratory” to find new, more efficient ways to reduce carbon emissions. Over time, he hopes solutions discovered at U of T could be rolled out in Toronto and other cities. “I believe we have the plan, the people and the drive to help Canada achieve its lower-carbon goals,” he says.
Harnessing the Earth for Heating and Cooling
Groundhogs, famously, hibernate during winter. They do this to conserve energy when food is scarce, burrowing into a den below the frost line. Staying underground provides protection from predators, but it also helps keep the animal warm. Even on the coldest days, the temperature below the Earth’s surface stays between about four and 10 degrees Celsius.
This simple fact serves as the basis for geoexchange technology, which U of T will begin to install later this year underneath King’s College Circle. In winter, special pumps will extract heat from below ground and transfer it to water circulating in deep vertical pipes, which then will be distributed to buildings nearby. In summer, the process is reversed. The pumps extract heat from the buildings and transfer it to the water in the pipes for use the following winter. Since the pumps are powered by electricity, very little carbon is emitted in the process.
For the King’s College Circle project, more than 400 cylindrical holes will be drilled into front campus to a depth of 250 metres,1 making it the largest geoexchange system in a Canadian city. (Set end to end, these tunnels would stretch from U of T’s downtown campus to Barrie,2 Ontario.) When it’s finished, the system will significantly reduce the university’s reliance on natural gas, saving an estimated 15,000 tonnes of greenhouse gas emissions annually.
Smaller geoexchange projects are proposed for U of T Mississauga and U of T Scarborough. A second St. George project has been approved for the field at Robert and Bloor streets, to heat and cool a new student residence planned for Spadina Avenue, just south of Bloor.
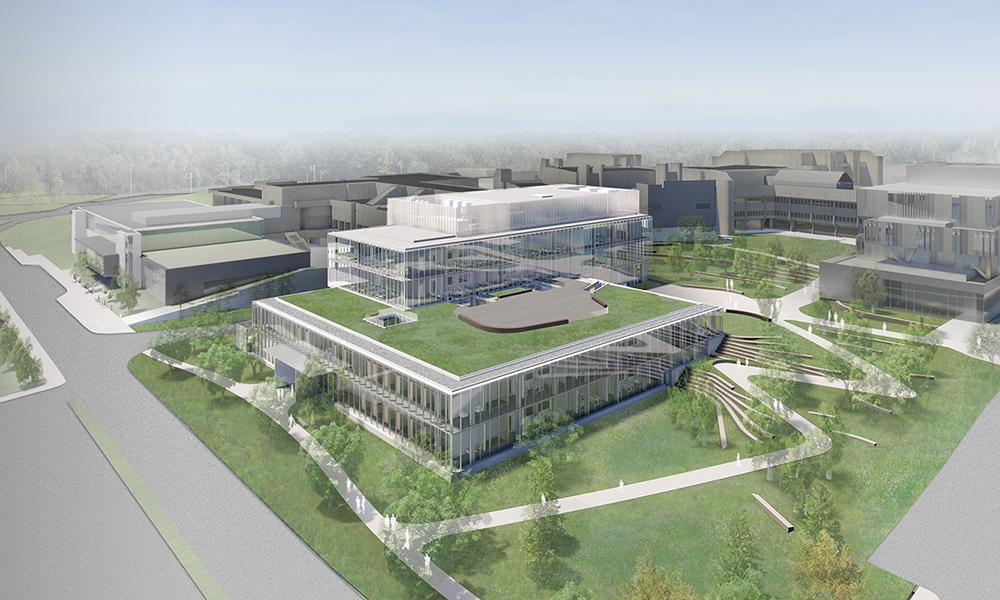
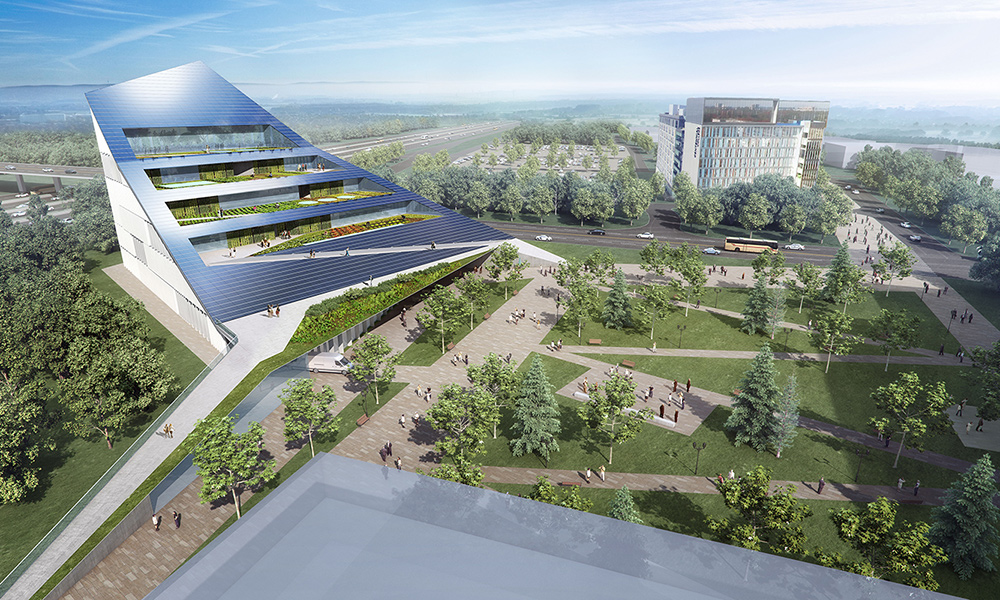
Raising the Bar for Green Buildings
Laboratories are known to be energy-intensive beasts: power-hungry equipment and 24-7 use don’t make for a model of sustainability.
UTM’s Science Building, slated for completion in 2023, will set a new standard for green design. The building – which will be home to the Centre for Medicinal Chemistry, the forensic science program, wet labs and a computing data centre – will be one of the most energy-efficient biological and chemical laboratory facilities in North America. It also exemplifies U of T’s more rigorous performance standards for new and renovated buildings. “Balancing the needs of a large research-intensive institution, while designing and constructing energy-efficient and sustainable buildings is imperative,” says Ahmed Azhari, the director of utilities and sustainability for UTM Facilities Management and Planning.
For most of its heating and cooling, the Science Building will use a geoexchange system and rooftop photovoltaics. Interior features include LED lighting throughout, automatic sash closing for fume hoods and real-time air-quality monitoring systems.3
When people think of a sustainable building, they might think exclusively in terms of a low-carbon footprint. But Azhari says there are three spheres of sustainability: environmental, social and economic – and U of T takes a holistic approach when it comes to new construction or major renovations. So, for example, the Science Building’s planned green roof and natural light wells will be not only environmentally friendly and money savers, but will also improve the experience and well-being of the people in the building. “Designing sustainable and environmentally responsible buildings is simply the sensible thing to do,” 4 he says.
Enlisting Students and Faculty to Help Advance Sustainability
U of T collects data on greenhouse gas emissions from its buildings, but no central report exists for emissions generated by the business travels of faculty and staff. Now, students in a course taught by John Robinson, a professor at the School of the Environment, are working to rectify this.
The effort is one of six “Campus as a Living Lab” projects being undertaken at U of T. The idea behind them, says Robinson, is to involve students and faculty in testing new sustainability programs. Other activities include evaluating the comfort and environmental performance of the Daniels Building at One Spadina Crescent, and examining how U of T’s guide for running sustainable events could be made more useful, and more widely used, across the university. In the future, a vertical farm slated to open at U of T Scarborough in 2023 will serve as an interactive lab for students and could even provide food for the community.
“This is a massive opportunity for the university to engage with problems in ways that are helpful to society but also incredibly helpful and popular with our students,” says Robinson. The goal is to have a thousand students a year working on Campus as a Living Lab projects, he adds. “That’s where we want to get to – where all new projects are deeply sustainable and they have a research and teaching dimension. We’re just starting this process.”
U of T Scientists Aim to Turn CO2 into Something Useful Before It Enters the Atmosphere
At the same time U of T is working to reduce the amount of carbon dioxide it releases into the atmosphere, several researchers are developing ways to convert the greenhouse gas into useful products before it ever gets there.
Scientists working in the labs of Dave Sinton, a professor in the department of mechanical and industrial engineering, and Ted Sargent, a professor in the department of electrical and computer engineering, have teamed up to build a system that uses low-cost renewable energy, such as wind and solar, to convert carbon dioxide and water into ethylene, ethanol and other chemicals. These serve as the building blocks for a variety of products that would otherwise be created from fossil fuels.
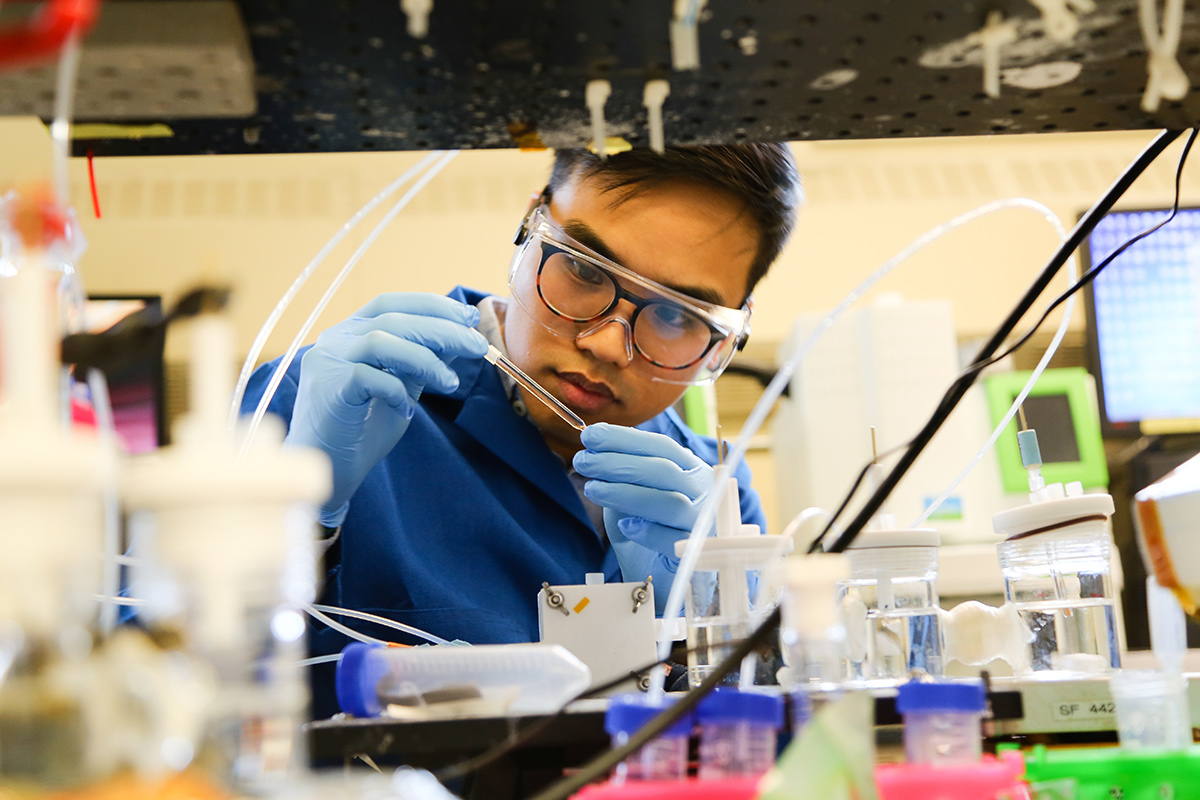
This technology is not a distant dream. The U of T team is building a test facility right now as it competes for the NRG COSIA Carbon XPRIZE, an international competition to develop carbon-conversion technology. Two winning teams will receive $7.5 million each, determined by how efficiently their system converts carbon dioxide into valuable products. Between now and June, U of T and the nine other finalists will demonstrate the viability of their technology at facilities in Alberta and Wyoming. “The judges will be looking at how many tonnes of carbon dioxide we can convert and the value of the products we make,” says Sinton.
Win or lose the contest, the U of T test plant will return home to serve as the foundation for a larger carbon dioxide conversion facility here 5 that develops various technologies emerging from university labs – and helps to further reduce net carbon emissions across the three campuses. Eventually, says Sinton, his team hopes its carbon-capture system could be deployed globally. “We’re excited to be planting these seeds at U of T.”
Beyond 2030, and the Drive to Carbon Neutrality
U of T’s Low-Carbon Action Plan gets the university most of the way toward its 2030 target. But what happens after that? U of T will still be emitting almost 75,000 tonnes of greenhouse gases a year, and many scientists believe that the world will have to be carbon neutral by 2050 to avert the worst effects of global warming.
So when Ron Saporta looks 30 years down the road, he’d like U of T to be leading, not lagging behind, the rest of the world.6
A new, longer-term commitment toward zero net emissions could be coming from U of T as early as this year, along with a roadmap for how to get there. Saporta estimates the university will have to invest hundreds of millions of dollars over the next three decades to achieve carbon neutrality. Calculated on an annual basis, though, he says this is manageable within the university’s budget.
There will be no one solution. U of T will have to draw on many new and existing technologies, such as air heat pumps, which could be used to draw energy from even cold air to heat a building efficiently. The university is looking at biomass (which uses organic waste to generate energy), electric vehicles and even recovering heat from the city’s sewer pipes.7 Of course, it’s almost impossible to predict what technologies may become available in the coming years, so the 2050 plan will have to be flexible. “We’re looking at everything,” says Saporta.
12 Responses to “ U of T’s Path to a Low-Carbon Future Begins Deep Below King’s College Circle ”
These are some wonderful innovations, but if these upgrades are being funded by income from coal and gas investments, does it really make us leaders? What progress has been made in convincing the university to divest from fossil fuels?
From Jacqueline Allain (BA 2015 New)
If U of T wanted to do its part to combat climate change, President Meric Gertler should have approved divestment from fossil fuels when he had the chance in 2016.
@Mira, Jacqueline
The University of Toronto is advancing research and education related to climate change and sustainability, and is committed to significantly reducing its own carbon footprint at its three campuses. As well, the University of Toronto Asset Management Corporation has assumed a prominent role in the global investment community, incorporating environmental, social, and governance factors into its investment decisions. These efforts are all part of the university’s multi-pronged approach to meeting the global challenge of sustainability. Read more here.
Amazing, forward-thinking innovations!
I am very pleased to know that you are reducing the carbon footprint at the U of T campuses.
What’s the total volume of the water in all the geothermal tubes? Have you considered the possibility of the water being overheated in summer? Steam build-up might wreak havoc, though to what extent I don’t know. The reverse should also be considered: frozen pipes during winter.
@Kam
Ron Saporta writes:
The temperature of the water in the geoexchange system typically falls between 10 and 27 degrees Celsius, changing seasonally. A heat pump in our proposed mechanical room would extract heat from this water in winter and reject it in the summer. The water inside the pipes remains in this temperature range, so there is little risk of ice or steam within the geoexchange system. As well, the pipes sit well below the frost line, and therefore are not at risk of being frozen during winter conditions.
The King's College Circle project looks to be impressive. However, the article doesn’t state the estimated construction cost. Also, what is the estimated annual cost saving for heating and cooling the buildings this project will serve? And what is the inside diameter of the 400 pipes to be installed?
@Richard
Ron Saporta writes:
Since this will be part of a public procurement process, the university does not reveal capital costs. The project will offset an estimated $1.5 million in annual operational expenditures. The diameter of the pipes will be 40 mm.
This is good news. However, we need lower carbon emissions standards for cities and institutions to meet our targets. Even net zero is not sustainable because there is so much heat already locked in to the planetary system. We need to be sequestering carbon rapidly.
Interesting and bringing hope...
These ideas represent a remarkable vision to lead the world in economic, environmental and social sustainability. I would love to collaborate to export these ideas and local technologies to address the challenges in Asia.