For decades, quantum computing research largely happened in university laboratories, where the field’s tremendous theoretical potential contrasted with the reality of slow, incremental progress. Today, though, startups and established tech companies are helping to drive advances in the field, bringing quantum computing into the mainstream.
One Toronto-based startup, Xanadu, is betting on a branch of quantum computing known as “photonics,” which harnesses particles of light, or photons, to manipulate quantum information. In its lab on the 29th floor of a downtown Toronto office tower, the company’s scientists work at dust-free tables chock-a-block with lenses, mirrors and fibre-optic cables, manipulating light at the quantum level to encode and process information. (Outside the lab’s entrance, the scientists have stationed a carton of disposable “booties,” which must be worn inside to keep the space pristine). In all, the company employs 50 people to design, test, refine and sell its light-based quantum computing system.
Adjacent to the lab, the company’s offices bear the telltale signs of startup culture: An open-plan workspace abuts a glassed-in room with a bright blue ping-pong table. Staff sometimes stick around after work to play board games or challenge each other on an arcade machine that runs classic games like Pac-Man and Space Invaders. “More than half of our employees moved to Toronto to join the company,” says Zachary Vernon (BSc 2012, PhD 2017), who works at Xanadu as a physicist. “The office has become like a second home.”
Growing interest in the potential for quantum computing to usher in a new era of digital advances has prompted a race for talent – and investors. Last October, Nature reported that, in recent years, private funders have sunk hundreds of millions of dollars into dozens of quantum technology startups. At the same time, some of the world’s largest tech companies, such as Google and IBM, have ramped up their own investments.
Xanadu, which was founded in 2016, recently secured $32 million in Series A financing, is developing both hardware and open-source software. Unlike many traditional computing businesses, though, the company has no immediate plans to sell devices or enter the consumer market. Instead, it will charge organizations for access to its photonics-based quantum computers through the cloud.
Who will buy? Some of the most obvious clients are those requiring advanced computation, such as banks and pharmaceutical companies. But Xanadu envisions selling a wide range of computing tools for use in fields that require certain kinds of complex calculations, such as drug development. “We see quantum chemistry as a key area,” says Nathan Killoran (MSc 2007), who works on software development for Xanadu. “We try to simulate the properties of a chemical system and then optimize the model to get better and better predictions.” Materials scientists could use these simulations to develop new materials, such as superconductors and industrial chemicals.
How quantum computers work is notoriously difficult to explain. They store and process information in atomic and subatomic particles or other ambiguous entities called “quantum objects.” The laws of physics at this incredibly minute scale should eventually enable quantum computers to outperform the world’s most powerful existing computers on certain tasks. Both startups and established tech companies are racing to achieve this milestone, which is known in the industry as “quantum supremacy.”
The term is ambiguous: last October, Google claimed supremacy for its quantum computer, named Sycamore, which in less than five minutes generated random numbers in a way the company said would take the world’s most powerful computers more than 10,000 years. But critics have argued that traditional machines could have done the calculations much more quickly than Google estimated, and that the task Google selected for Sycamore was too specific and esoteric to be meaningful.
While today’s conventional computers harness billions of binary bits to do their calculations, Google’s Sycamore ran on 53 “qubits” – a basic unit of quantum information that’s analogous to a classical bit. Most modern classical computers store bits in tiny transistor switches on a microchip. With a qubit, quantum information can be stored in the spin of an electron, in the direction of electricity flow in a superconducting circuit (at ultra-low temperatures), or in tiny semiconductors called quantum dots, as well as in particles of light. Each method has advantages, disadvantages and unique qualities that make them better suited for different tasks. The kind of quantum devices Xanadu is developing rely on photons, which have more quantum states and can therefore store and process more information. “Photonics is a premium way to communicate information,” says Killoran. “Their specific quantum properties support a unique set of applications.”
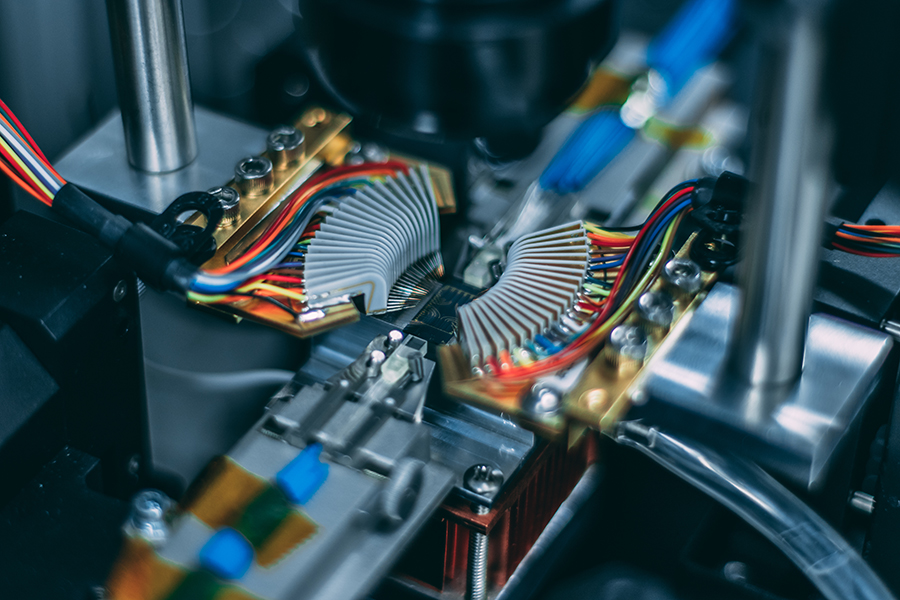
While scientists and entrepreneurs debate the relative merits of different systems, most agree on the general level of complexity required for quantum supremacy. “It’s pretty clear that once you get past a few dozen qubits, certainly if you’re at one hundred, then you’re past the point where you might be quibbling over the details of what, from a very academic perspective, counts as supremacy,” says Vernon.
But even the simplest, most robust quantum systems face challenges having to do with as-yet-unanswered fundamental questions of physics. Quantum computing requires that information pass from the “classical” world of our everyday existence into the quantum world of subatomic particles. But quantum and classical physics have different rules, and no scientist fully understands the relationship between the two.
To address these challenges, companies are vying for highly specialized talent in a relatively small pool. Thirty-one of Xanadu’s 33 scientists hold PhDs in physics. Founder Christian Weedbrook, who developed the company’s business concept four years ago before entering the university’s Creative Destruction Lab, did a postdoctoral research fellowship in quantum physics at U of T.
Weedbrook believes that photonics represents one of the most viable avenue for overcoming the initial hurdles of quantum computing: it’s comparatively simple to move information in and out of a photonic system, and potentially easier to network photonic qubit arrays, which allows them to build up to higher levels of complexity. Photons are also more stable than many other quantum particles. And, because they work at room temperature, photonics systems are easier to modify and upgrade. In fact, they use fibre-optic cables and many other conventional components that are already mass produced.
With investors and entrepreneurs increasingly viewing quantum computing as a business and investment opportunity, researchers talk more assertively about the field’s progress so far, as well as its near-future potential. “There are a lot of parallels with the development of traditional computers 50 years ago,” says Killoran. “Some people say we’re still very early in the vacuum-tube era. I think we’re further along. I’d say we have the transistor, and we’re starting to show some proof of concept in the microprocessor. We’re around the equivalent of the early 1970s.”
And as quickly as computing technology has advanced since then, Vernon says that quantum computers could progress even faster. “A lot of the technological maturity of the microelectronics industry can be borrowed and used for quantum,” says Vernon. “We don’t need to reinvent the wheel.”
As the reality of quantum computing begins to catch up to the potential, the business environment becomes increasingly competitive. Weedbrook, though, trusts that his company is on the right track. “We try to focus on what we can control and not on what others are doing,” he says. “We love what we do, and we learn each day. If those two things are happening, we are increasing our chances of success.”
How We Arrived at Quantum Computing
We’re approaching the limit of classical computing power. The quantum realm opens up new possibilities
Every traditional computer, no matter how sophisticated, runs on simple binary digits (zero and one), or “bits,” which control switches with two possible states – on and off. A computer with more bits can do more calculations more quickly. Switches turn on and off to perform mathematical calculations, activate pixels on a screen, encode and decode information, and do every other task we demand of our devices.
Breathtaking advances in computing power over the past several decades has revolved around shrinking these switches. In 1946, University of Pennsylvania scientists completed work on the first fully functional, programmable digital computer, which used 18,000 bulky vacuum tubes for switches, weighed nearly 30 tons, and covered about 1,800 square feet. The next generation of computers used smaller transistors as switches. Transistors gave way to integrated circuits, the electronic components of modern microchips. Today, a single chip can house billions of transistors in an area the size of a thumbnail.
Switches can only get so small, though, before they hit the dividing line between classical physics and the quantum realm. When researchers start storing and processing information in quantum systems, the switches don’t just get smaller – the very nature of physics and information change entirely.
A quantum bit can be on and off at the same time. A qubit can actually occupy many “states” simultaneously. Adding qubits to a system increases the number of possible states exponentially. That’s why a quantum computer with just 100 qubits could theoretically take on some tasks that are impossible for classical supercomputers, with billions of bits. In practice, though, quantum systems are delicate and prone to disruption and error. Advances in the past five years have focused on creating more stable, manageable systems that are easier and cheaper to build, with better error correction, and greater flexibility and robustness.
Recent Posts
People Worry That AI Will Replace Workers. But It Could Make Some More Productive
These scholars say artificial intelligence could help reduce income inequality
A Sentinel for Global Health
AI is promising a better – and faster – way to monitor the world for emerging medical threats
The Age of Deception
AI is generating a disinformation arms race. The window to stop it may be closing
2 Responses to “ As Quantum Computing Moves from Theory to Market, the Race for Supremacy Heats Up ”
This is so fascinating to read -- and contemplate other potential uses. I’m a U of T grad and a vascular and skull base neurosurgeon. One of our challenges is to decipher which aneurysms -- weaknesses in blood vessel walls -- will eventually rupture and potentially kill one-third of the patients affected. Wouldn’t it be nice to use quantum physics to look into vessel-wall abnormalities and wall-motion abnormalities to predict which flow turbulence patterns precede an imminent rupture? I’m certain that certain structural defects lead to different turbulence patterns. Quantum physics and AI could help develop a predictive model that would help guide the treatment paradigm in this deadly disease.
This is fascinating stuff. Please keep me in the loop.